Table of Contents
Introduction
In the realm of physics, electrostatic fields hold tremendous potential and play a significant role in our everyday lives. From the sparks created by rubbing a balloon against hair to the complex systems that power our technology, understanding electrostatics is essential.
In this blog post, we will dive into the captivating world of electrostatic fields, exploring their fundamental principles, real-world applications, and various questions related to the electrostatic fields such as whether the electrostatic field is conservative.
The Basics of Electrostatic Fields: Electrostatics and Electric Charges
Electricity is a fundamental aspect of our daily lives, and electricity is produced from the interaction between electric charges. Electric charges can be classified as positive, negative or neutral. Protons are associated with positive electric charges, while electrons are linked with negative electric charges.
Neutral electric charges, conversely, are electrically balanced and have no excess of either negative or positive charges. In situations where an object has an equal number of positive and negative charges, they cancel each other and result in a net neutral charge. Electric charges are quantized, meaning they occur only in discrete values known as elementary charges. The nature and behaviour of electric charges are critical to understanding electricity, as they determine the flow of electric current through conductors.
The concept of charge conservation
The concept of charge conservation plays a fundamental role in various branches of physics and engineering, as it states that the total electric charge in an isolated system remains constant over time. This principle is based on the conservation of charge, which means that charge cannot be created nor be destroyed, it is only transformed from one form to another.
By understanding and applying the concept of charge conservation, scientists and engineers can accurately predict how electric charges will behave in different systems. This understanding is crucial in fields such as plasma physics and beam physics, where the simulation of Maxwell’s equations with charge is essential. Additionally, charge conservation is also important in the study of biochemical systems.
The electrostatic field is conservative or not
A conservative electrostatic field exists. The electrostatic field is conservative, according to the basic theories of electrostatics. This indicates that a charged particle’s work on an electrostatic field is independent of the direction the particle travels.
Furthermore, the electrostatic field satisfies several relations, such as the relation between the electric potential and the field of a point charge. Since the electrostatic field is a conservative force field, any work it performs on a charged particle is independent of its route.
Mathematics of Electrostatics
Coulomb’s Law:
Coulomb’s law describes the force between two point charges. It says that the force (F) between two charges (q1 and q2) is inversely proportional to the square of the distance (r) between them and directly proportional to the product of their magnitudes. In mathematics, Coulomb’s law is expressed as:
F = k * (q1 * q2) / r^2
Here, k is the electrostatic constant, approximately equal to 9 × 10^9 Nm²/C².
Electric Fields:
Electric fields are a useful metaphor for explaining how charges affect their surroundings. A unit positive charge placed at a point in space will feel a force called an electric field (E) there. The following formula can be used to determine the electric field:
E = F / q
Where F is the force experienced by the charge q due to other charges in the vicinity.
Gauss’s Law:
According to Gauss’s law, the charge distribution on a closed surface and the electric field are related. It asserts that the total charge encapsulated (Q) divided by the permittivity of space (ε₀) equals the total electric flux (ΦE) through any closed surface.
ΦE = ∮ E · dA = Q / ε₀
The integral symbol (∮) denotes the surface integral, E represents the electric field, dA is an infinitesimal vector area element, and ε₀ is the permittivity of free space (approximately 8.85 × 10^(-12) C²/Nm²).
Electric Potential (V):
The electric potential energy per unit charge at a specific site is represented by the scalar quantity known as electric potential (V). The labour required to move a unit positive charge from one point to another is equal to the electric potential difference between two points.
V = -∫ E · dl
Where E is the electric field vector, dl is an infinitesimal displacement vector along the path of integration, and the negative sign indicates the direction of the electric field lines.
Capacitor:
Two conductive plates are separated by a dielectric material to form capacitors, which are used to store electric potential energy. A capacitor’s capacity to store charge is measured by its capacitance (C). The following formula can be used to determine it:
C = Q / V
Where Q is the charge stored on the capacitor and V is the potential difference (voltage) across its plates.
We can calculate the behaviour of electric charges and fields thanks to the mathematical foundations that make up electrostatics. They are crucial to the resolution of issues about electromagnetic interactions, electrical circuits, and several real-world applications in disciplines including engineering, physics, and electronics.
Experiment of Electrostatic field
Here, is an experiment that you can conduct to observe and understand the properties of electrostatic fields.
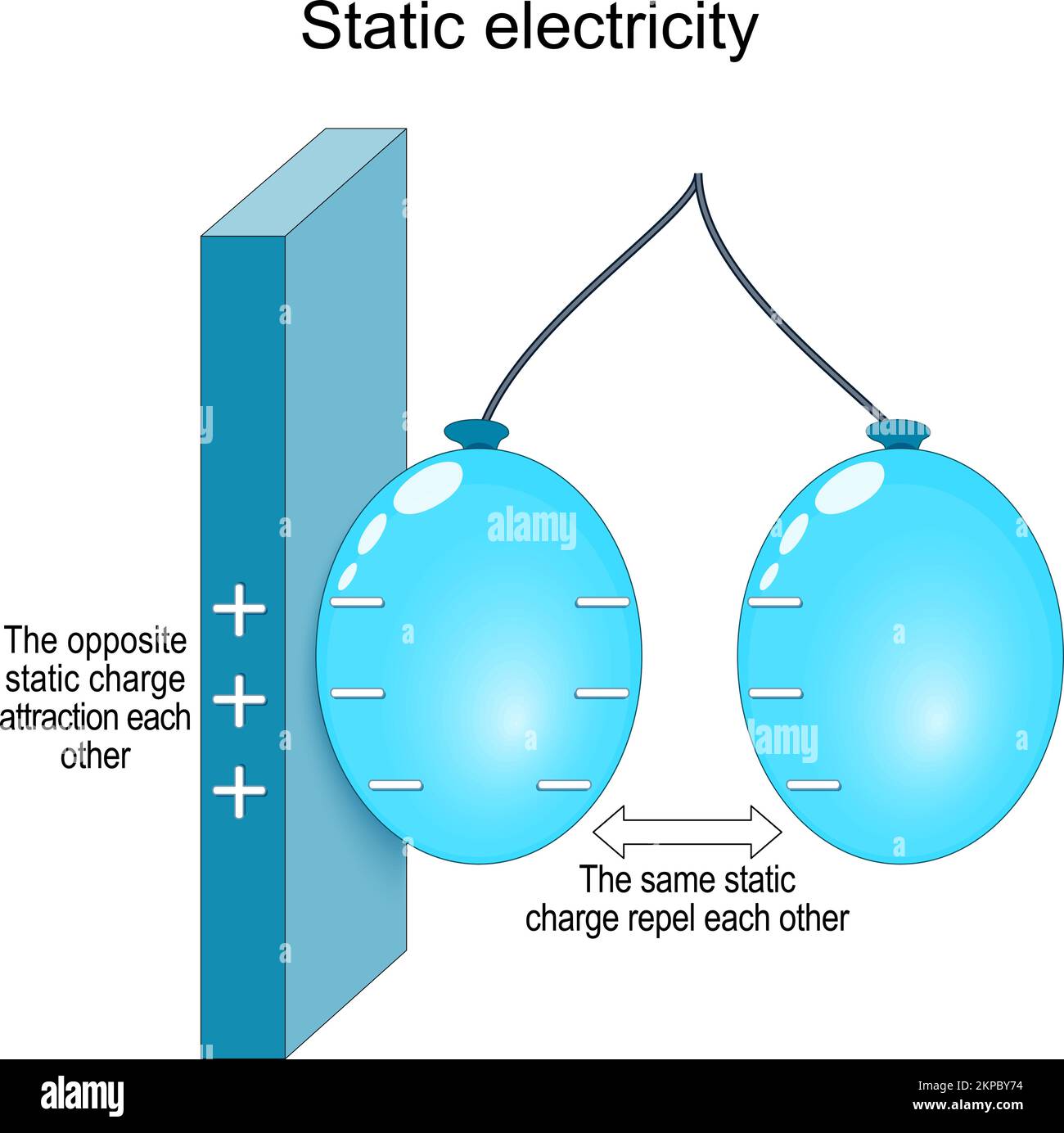
Objective:
To demonstrate the presence and behaviour of electrostatic fields using everyday materials and observe their effects.
Materials:
- Balloon
- Hair or fur
- Small pieces of paper or confetti
- String or thread
- A dark, non-conductive surface (such as a table or sheet of paper)
Procedure:
1) Prepare by blowing up the balloon and tying it tightly.
2) Trim a 30-cm-long piece of string or thread, and fasten one end to the balloon.
3) Charging the Balloon:
a. For around 30 seconds, rub the inflated balloon against some of your hair or some fur. By doing this, electrons from your hair or fur are transferred to the balloon, negatively charging it.
b. As an alternative, you can charge the balloon by rubbing it against other things like a wool cloth or another latex balloon that has been inflated.
4) Place the charged balloon on the non-conductive surface to see the electrostatic effects.
5) Suspend the balloon by holding the string or thread loosely.
6) Bring tiny pieces of paper in proximity to the charged balloon without touching it to attract objects.
7) Track the behaviour of the paper. They should attach to the balloon’s surface and draw attention to it.
8) Hold the charged balloon close to a neutral object, like a wall or another balloon that hasn’t been charged, to repel objects.
9) Watch how the neutral object behaves. You should notice it being repelled because electrostatic repulsion occurs when an object’s positive charge is caused by the balloon’s negative charge.
10) Exploring Field Strength:
a. Slowly move the charged balloon towards or away from the things being drawn to or repelled by the field.
b. Track how the items’ behaviour changes depending on the strength of the electrostatic field. Keep track of any changes in repulsion or attraction.
11) Experimenting with Different Materials:
a. Repeat the experiment using various materials (such as fur, wool, or various types of cloth) to charge the balloon.
b. Compare the findings and take note of any variations in the electrostatic field’s strength or behaviour.
12) Safety precautions:
a. Keep the experiment away from combustible or delicate electronic equipment.
b. Prevent the charged balloon from coming into contact with any liquids or water.
This straightforward DIY experiment allows you to see the effects of electrostatic fields up close. The charged balloon exhibits an electric field by attracting or repelling items. This experiment stimulates further research into this intriguing subject of science by allowing you to study the behaviour and strength of electrostatic fields.
Questions and Answers
What is an electrostatic field?
An electrostatic field is a type of electromagnetic field that surrounds an electrically charged object. This field exerts a force on charged particles in the vicinity of the object, either attracting or repelling them depending on their charge polarity. Additionally, the electrostatic force is a non-directional, long-range force and is an important phenomenon in the study of interactions at the molecular and nano scales. The electrostatic field is derived from the electron momentum equation for a non-equilibrium stationary state, which includes contributions resulting from the collisional transfer between electrons and protons.
How electrostatic field is produced?
The electric field, also known as the electrostatic field, is a fundamental concept in physics that describes the influence of electrically charged objects on each other. It is produced by any distribution of electrical charge, whether that’s a single object with an overall positive or negative charge or a more complex system of charged objects.
Whenever there are charges present, the electric field is produced around them. This field is created due to the forces generated between positive and negative charges, which may attract or repel one another. As a result, charged particles will tend to move toward areas of opposite charge or away from areas of like charge, creating an electric field that permeates throughout the surrounding space.
Why electrostatic field is zero inside a conductor?
When an electric charge is applied to a conductor, it spreads out evenly throughout the entire surface of the conductor due to its internal repulsive forces. As a result of the even distribution of charge, there is no accumulation or concentration of charges within the conductor. This leads to the creation of an opposite-field inside the conductor that cancels out the original electric field. Therefore, the net electrostatic field inside a conductor is zero.
Does the electrostatic field depend on mass?
Electrostatic fields are generated by charges at rest and affect other charged particles in their vicinity. Therefore, electrostatic fields do not depend on the mass of particles. Electrostatic fields are generated by charges at rest, and they do not depend on the mass of charged particles.
Conclusion
Electrostatic fields continue to captivate scientists, engineers, and enthusiasts alike. From the fundamental principles that govern them to the applications they enable, electrostatics plays a crucial role in numerous areas of science and technology. As we continue to unravel the mysteries of electrostatic fields, the potential for groundbreaking innovations and discoveries is limitless. So, let us embrace the power of electrostatics and embark on a journey of electrifying possibilities.
1 thought on “Electrostatic field is conservative or not”